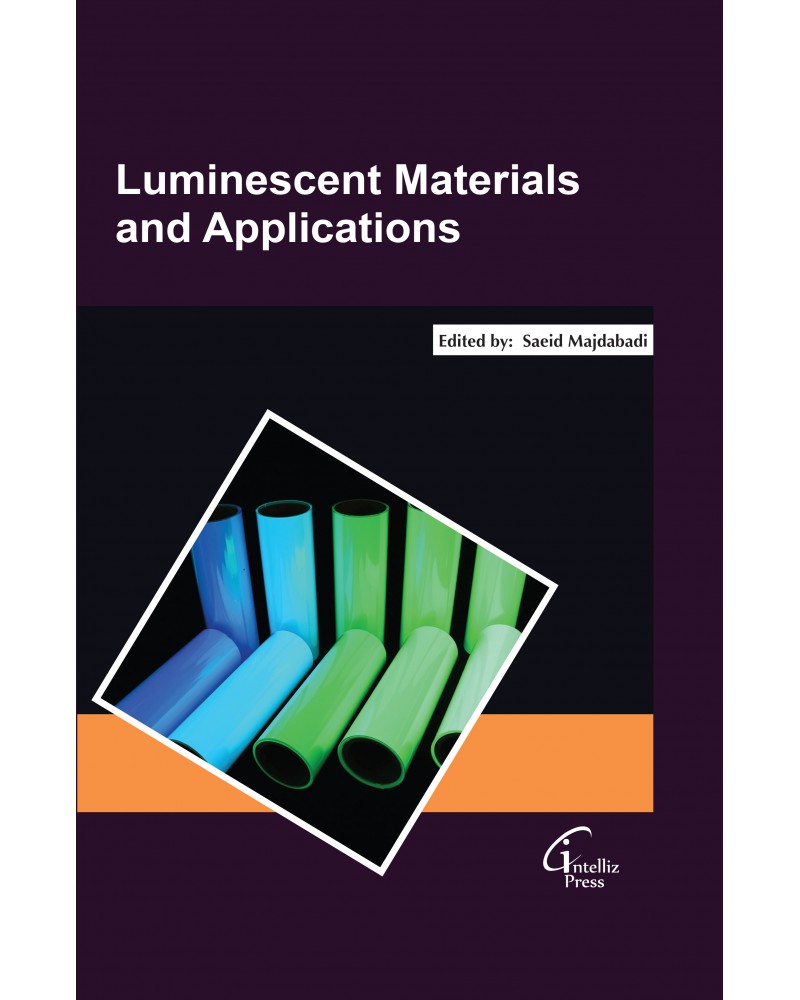
What Are the Different Types of Luminescent Materials?
What Are the Different Types of Luminescent Materials?
There are a number of different types of luminescent materials. These include rare earth-doped luminescent materials, inorganic phosphors, and photon upconverting materials. They are used in a wide variety of applications from lighting to astronomy. However, it is important to understand how they work in order to be able to use them properly.
Inorganic phosphors
Inorganic phosphors are luminescent materials, which convert energy into electromagnetic radiation. They are commonly used in a variety of applications. These include light-emitting diodes (LEDs), fluorescent lamps, and X-ray detectors. The emission of these phosphors depends on the host material and the nature of the ion.
When an inorganic phosphor is excited, part of the excitation energy is transferred to the host lattice. This absorption and decay can be controlled by controlling the temperature and defects.
In the present disclosure, we describe luminescent materials that are doped with rare-earth ions. These ions affect the properties of the materials and improve their performance. Rare-earth ion-doped inorganic phosphors have practical applications in many devices, including LEDs, fluorescent lamps, and X-ray detectors.
Using a sol-gel method, europium (III) and strontium silicate (Sr2SiO4) phosphors were synthesized. The Eu3+ site was crystalline, while the Sr2SiO4 site was less symmetric and had a bandgap property. Using a general structure analysis system, we determined the weight percentages of the a’- and b-SSO phases.
Our results revealed that the a’-SSO phase had a mixed orthorhombic structure. It consisted of a b-SSO phase with a monoclinic angle of th and an a’-SSO phase. We also found that the crystal angle th was reduced in the b-SSO phase. However, the a’-SSO phase was still present.
Our study suggests that the emission peaks of europium (II) depend on the host lattice. This results in a narrow line of emission. Moreover, it allows for the transfer of energies between different levels of the ion. X-ray emission spectroscopy and synchrotron-based X-ray absorption near-edge structure were used to analyze the relationship between the PL emissions and the local structures.
The results showed that the phosphors exhibited a red PL spectrum. Specifically, we observed that the emission of the Er3+ ions was responsible for the red emission at 666 nm. Also, a green emission at 548 nm was found.
The morphology of the particles is controlled by a sol-gel procedure, which has high chemical homogeneity. Moreover, the O2- charge-compensating agent occupies the sites of defects. Therefore, this technique can be employed to develop ordered oxyfluoride compounds.
Rare-earth-doped luminescent materials
Rare earth-doped luminescent materials are highly versatile materials with a wide range of applications. These include solid state lasers, plasma televisions, and light-emitting devices like fluorescent lamps and liquid crystal displays. The high optical quality, thermal stability, and luminescent materials chemical properties of rare earth based nanomaterials have attracted researchers to explore the potential of these materials.
Dopant materials are produced by applying a reactive atmosphere treatment to a multicomponent starting material. This process produces water-insoluble hydroxide precipitates, which can be subsequently transformed into nanoparticles. By judicious choice of dopant ions, the photophysical dynamics of these materials are greatly improved.
Rare earth elements are very efficient activators. They can emit red, green, and blue light. In addition, rare earth metals and fluorides can convert near-infrared light to UV/visible light. Their fluorescence emission is also strong in the orange light region.
The rare earth ion(s) doped luminescent materials are one of the important classes of nanophosphors. Their use has led to the development of a new telecommunications superhighway, as well as many other technological advancements. Moreover, their application in medical imaging and drug delivery is common.
Research in rare earth-doped oxide hosts has increased rapidly in recent decades. Due to their intense luminescence and broad band, these compounds have a strong potential for applications in visible-display technologies.
Moreover, rare earth-based nanomaterials can be synthesized at nanoscale. Luminescent materials can be used for white and multi-color illumination in various applications. Since their applications are wide-ranging, they have attracted great research interest.
The demand for advanced phosphors is increasing due to the development of display technology. This research focus has led to the development of highly doped materials of the present invention. Compared with conventional luminescent materials, the highly doped materials exhibit broader absorption, broader emission band, and improved transfer and reception of infrared signals.
The present invention is applicable to a variety of hosts, including halide salts. Halide salts contain weaker bonds than oxide-based compounds. Because the bonding strength is lower, the influence of the host on the luminescent ions is reduced. Moreover, because the host has a low phonon energy, applications using a relatively small consumption of halide materials are possible.
Photon upconverting materials
Photon upconverting materials are a class of materials which absorb and emit two low energy photons into one high energy photon. They are found in a wide range of applications, including display technologies and sensing. However, there is a lack of commercial instruments to measure their performance. Consequently, collaborations between researchers are essential. In order to find a wider distribution and improve their performance, more research is needed.
The upconversion efficiency of materials depends on the particle size, surface geometry, and material. Some materials exhibit narrow excitation bands. It is important to control the absorbed and emitted wavelengths for efficient upconversion. For this purpose, photon upconverting nanoparticles (UCNPs) can be used. UCNPs are small homogeneous lanthanide doped nanocrystals which usually contain an organic solvent.
Using a hydrophilic ligand as a growth-controlling reagent helps reduce the particle size and increase the surface-to-volume ratio. A variety of surface modification methods have been developed for UCNPs. These include modifying the surface with a functional group, such as a peptide, and using a small ligand, such as azelaic acid, to suppress quenching.
Other surface modifications rely on non-covalent interactions, such as thulium (Tm3+), which can produce distinct emission bands. Similarly, the addition of gold nanoparticles can improve the upconversion intensity.
Surface modification also affects colloidal stability. The size of the ligand and the nature of its quenching factor are critical in determining the upconversion efficiency. There are a variety of ligands, including polyacrylic acid, which is large and bulky, and azelaic acid, which has a smaller ligand and differs in its quenching factor from water.
Optimal surface ligands must be cytotoxic, biocompatible, and have a reduced surface quenching effect. To achieve maximum upconversion efficiency, UCNPs should be synthesised with a well-defined, aqueous colloidal dispersion. Moreover, they should be designed carefully in terms of both the surface and the particle dimensions.
Nanoparticles based on UCNPs have potential for background-free imaging. Their narrow absorption spectra make them ideal for detection of trace amounts of target molecules. Although UCNPs are currently not commercially available, they have a lot of promise for biosensing applications.
Observing a light stick’s luminescence
If you are studying chemiluminescence, you can conduct an experiment to see what happens when a glow stick is heated. You can also observe other objects glowing when exposed to a black light. This lesson will help you to understand what luminescence is and how it works.
After observing a light stick, you can compare the properties of the sticks. For example, you can find out how long it takes for the stick to glow. Also, you can determine how bright it is. To do this, you can start one stick in cold water and immerse it in hot water. As the temperature of the water increases, the light will become brighter.
You can also place a light stick in water. This is a good way to observe how a glow stick’s brightness changes over time. However, be careful to make sure that the plastic is not melting.
Before you do this, you should have the participants wash their hands. They should then record their observations of the stick. Some of the things you might want to include are how it is shaped, how it emits light, and what it can be used for.
After you have finished the task, you can write a prediction about how the stick might glow. Then, you can describe the type of luminescent materials chemical reaction that causes it to glow.
It is important to remember that safety is paramount when conducting any experiment. Make sure to wear the appropriate safety equipment and follow all manufacturer’s instructions. In addition, you should always use proper laboratory procedures.
One way to do this is to conduct an experiment in which you start three glow sticks in different temperatures. Then, you will determine how the temperature affects the rate of the reaction.
You can even use a digital camera to record how the sticks are shining. When the digital camera is recording, you can try to capture an image that shows the glow of all three sticks. Finally, you can create a chart with columns for each type of luminescence. By doing this, you will be able to identify each column and label it.